Research
Elucidating drug resistance in multiple myeloma using peptide-based biosensors and droplet microfluidics.
Patients diagnosed with multiple myeloma have been found to either respond or not respond to single-drug therapeutics that target the proteasome. This has led to treatment protocols using quadruple drug therapies to increase median patient survival. Currently, it is unknown why some patients respond to single vs. multi-input therapeutics. The scope of this research is to gain a better understanding of the mechanism of drug resistance in multiple myeloma by developing cell-permeable, long-lived, fluorescent peptide-based biosensors to measure both proteasome and deubiquitinating enzyme (DUB) activity in single intact cells. A hallmark of our biosensor is a β-hairpin sequence motif that functions as both a cell-penetrating peptide (CPP) and protecting element to prevent protease-mediate cleavage of the reporter increasing intracellular lifetime.
Single-cell measurements are essential to identify the drug-resistant subpopulations that oftentimes drive relapse in cancer patients due to cancer heterogeneity. Single-cell measurements are made possibly by the use of a droplet microfluidic trapping array capable of isolating and performing dynamic analysis of changes in enzyme activity in response to proteasome and/or DUB-based therapeutics. The high-throughput analysis is made possible by custom Python code capable of analyzing hundreds to thousands of cells in a matter of minutes. We use clustering algorithms with our collaborators to elucidate this data to identify drug-resistant versus drug-susceptible populations based on changes in proteasome and DUB activity.
Studying drug resistance and tumor progression in breast cancer using engineered 3D cell culture.
Cells cultured as a 2D monolayer have been demonstrated to exhibit differences in phenotype when compared to 3D cultured cells. Moreover, many drugs that show success in the 2D environment fail to kill cancer cells in vivo due to the inherent mass transfer limitations of the drug into the tumor. As such, there is a need for model systems to better recapitulate the 3D tumor microenvironment. This research area uses 3D breast cancer spheroid as a model system to study intracellular signaling and drug resistance in ER+ breast cancer. Our unique approach utilizes a thiol-acrylate (TA) hydrogel scaffold coupled with a droplet microfluidic trapping array to perform high-throughput screening of hundreds of 3D spheroids. The TA scaffold is unique in that it degrades over time allowing the spheroids to ultimately generate their own matrix and allow for on-chip immunostaining. Our approach also allows for the co-culture of cancer, immune, and stromal cells (e.g., adipose-derived stem cells and fibroblasts) in the spheroids to account for direct and indirect cell-to-cell communication in the tumor microenvironment. These multi-cell type spheroids are being used to study how paracrine signaling between ASCs and fibroblasts alters the efficacy of endocrine therapies in estrogen receptor-positive (ER+) breast cancer.
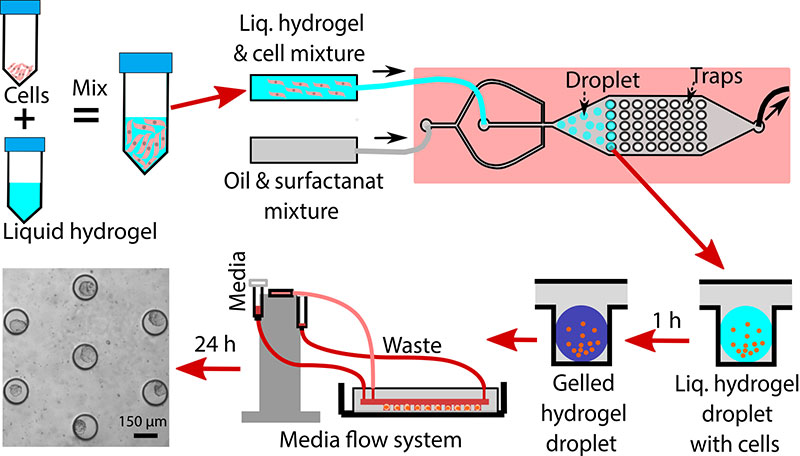
Evaluating tumor/stromal/immune cell-to-cell communication in the breast tumor microenvironment with new co-culture techniques.
The tumor microenvironment is a complex system comprising of multiple cell types including stromal, immune, and cancer cells. Recent research has identified that paracrine signaling between these cell types can drive both cancer proliferation and drug resistance. We developed a microfluidic co-culture platform that showed that adipose-derived stem cells (ASCs) induce drug resistance in triple-negative breast cancer. Our current research efforts focus on expanding these studies and elucidating the underlying mechanisms driving cancer progression and de-sensitization to therapeutics. To accomplish this, we have developed two unique approaches to co-culture two different cell lines in a single platform. One approach uses a 3D-printed plate insert for 10 cm dishes and 6-well plates separated by an agarose slab to facilitate the mass transfer of biomolecules. This approach allows for facile harvest of cell pellets for both PCR and Western blotting. The second approach involves a microfluidic device coupled with a peristaltic pump to allow for dynamic sampling of extracellular media to identify secreted biomolecules by the different cell types involved in paracrine signaling. The microfluidic approach also allows for direct visualization of cells to inspect morphology and terminal immunostaining to elucidate intracellular signaling pathways. Using these devices, we are studying how cancer cells communicate with stromal cells, immune cells, and even tissue-resident bacteria as part of the breast tumor microbiome.
Investigating how exposure to fluid shear stress (FSS) during metastasis alters breast cancer phenotype and genotype.
During metastasis cancer cells experience various magnitudes and durations of fluid shear stress (FSS). This pressure applied to the cell can result in both cellular deformation and phenotypic/genotypic changes. Moreover, several studies have shown that metastatic cancer tends to be more aggressive and drug-resistant when compared to the primary site. This research area focuses on studying how FSS alters cancer cell behavior using a modular microfluidic approach. The system consists of two devices: a serpentine shearing module to deliver uniform magnitudes of FSS to individual cells and a microwell trapping array to collect the cells after shear and interrogate them. This approach allows for both on-chip immunostaining and off-chip evaluation of changes in gene and protein expression via PCR and Western Blot. We are currently exploring the underlying mechanisms of how exposure to FSS enhances the proliferation and extravasation of ER+ breast cancer.
Characterizing a new class of stable cell penetrating peptides (CPPs) incorporating a β-hairpin sequence motif.
Cell-penetrating peptides (CPPs) have garnered significant attention in recent years as an effective strategy to deliver drugs, probes, biosensors, and other cargoes into intact cells. One challenge with many unstructured peptides is their relatively short lifetime in cells due to their rapid cleavage by peptidases and proteases. To overcome this, we have developed a suite of long-lived CPPs containing either a β-hairpin sequence motif or D-chirality amino acids. Both peptides showed enhanced stability and rapid uptake in the cells. We have also demonstrated how the identity of a cargo conjugated to CPP can affect its internalization potential. We are currently continuing this work with an emphasis on elucidating the underlying mechanisms driving the uptake of the β-hairpin containing peptides with and without a cargo.
Development of long-lived PROTACs using a β-hairpin sequence motif.
Proteolysis targeting chimeras (PROTACs) are an exciting therapeutic alternative. Instead of blocking the active site of a target, PROTACs take advantage of the naturally occurring ubiquitin-proteasome system (UPS) to selectively degrade target proteins. This approach has the potential to treat some of the notoriously difficult ‘undruggable’ targets. One limitation of peptide-based PROTACs is their rapid degradation due to their lack of a secondary structure. We recently identified a new class of PROTAC that utilizes a β-hairpin sequence motif which functions as a degron, CPP, and protecting element to facilitate the ubiquitination, cellular uptake, and enhanced intracellular lifetime. Ongoing research is focused on leveraging the β-hairpin sequence motif to develop a new PROTAC targeting PTP1B, a key player in diabetes and insulin signaling.
Analyzing the collected and single cell migration behavior of the model algal species C. reinhardtii.
While cell migration has been extensively studied in numerous model organisms, little is known about the mechanisms driving the chemotactic response in algae. Recent work has shown that C reinhardtii utilize collective migration during chemotaxis in response to an ammonium gradient. To expand on this, we have developed two different microfluidic devices to study the collective migration of algae to various combinations of chemical gradients (e.g., nitrogen, phosphorous, or bicarbonate) or the single-cell swimming of algae under different environmental conditions. Microfluidics allows for the precise control of the gradient coupled with the ability to track single-cell migration to better elucidate algal swimming behavior and how they respond to extracellular gradients. These findings have implications in both harmful algal blooms (HABs) and algal biofuels.